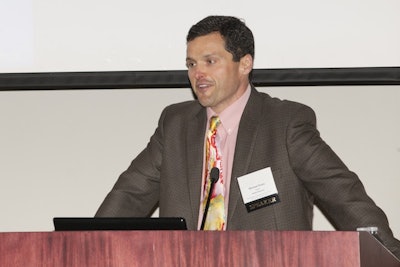
In part II of this updated column, I discuss a new business model for medical devices, and some of the regulatory challenges—both of which are as vital as the technology itself to make 3D printing a reality in medicine. We conclude with a look at the true potential of this technology – the ability to ‘print’ implantable medical devices, drugs, and even living tissue on-demand!
In my last column, I reported on how three-dimensional printing is already being used to produce medical devices. Yet just like pharmacogenomics, if we cannot “sell” the business model, i.e., make it palatable to the medical device industry, then no matter how “wicked cool” the technology is, it will never succeed.
The global market for 3-D printing in medical applications is projected to reach $965.5M by 2019. In comparison, the market was valued at $354.5M in 2012 with an expected to grow at a compound annual growth rate of 15.4% from 2013 to 2019 (Transparency Market Research, Dec. 2013). But this is just the tip of the iceberg—these numbers don’t include many of the applications I describe here.
Consider this: home-based healthcare has been a growing trend for years. So when, not if, patients can 3-D print their own medical devices at home, how many medical devices will device manufacturers be selling?
Maybe the medical device industry needs to consider a new business model. That is, instead of selling medical devices pre-sterilized in separate packages, they sell medical device designs that can be input into a 3D printer, or “prescribed” by a physician just like a drug. Both business models can be successful, but they are very different. What’s the first step in making this model a reality? To begin to think differently! Can you name one technology that existed prior to someone coming up with the idea and developing it?
Beyond the technical opportunities and challenges that these technologies offer, the regulatory implications are in many ways even more intriguing. Although the details are beyond the scope of this current column, recently the U.S. Food and Drug Administration released, “Paving the way for personalize medicine: FDA’s role in a new era of medical product development.”viii Of greatest importance is that this is the first major public document from FDA that begins to address personalized medicine from both a pharmaceutical as well as a medical device perspective.
Taking the regulatory concerns even further, consider this question: What is the relationship between 3D printing medical devices and pharmaceutical compounding?
For those who read my August column “The Case of the New England Compounding Center: How might this tragedy lead to an even bigger one," I explored the root cause of the NECC case and suggested that if we are not careful when crafting new regulation to prevent such tragedies from occurring again, we may simultaneously inhibit seemingly dissimilar technologies that have yet to be developed. On the surface, the NECC case would seem to impact only the compounding industry; but the ramifications could go far beyond compounding and drastically affect the medical device industry in ways most people have yet to imagine.
With that insight, personalized medicine and pharmaceutical compounding are really one and the same. And now with the ability to 3D print personalized medical devices at home looming, the lines dividing these technologies are becoming even more fuzzy—all the more reason we need to tread cautiously when creating new regulation. As I’ve discussed before, it’s one thing to measure the number of people harmed or killed because a medical product is not “safe enough”—it’s quite another thing to count the number of people harmed or killed because they don’t have access to a medical product because we’ve raised the “regulatory burden” to the point the manufacturer decides it’s just not worth bringing the product to market.
Looking to the future, one of the greatest limitations of 3D printing technology is the limited selection of usable materials. Historically, the materials used by 3D printers have been relatively rigid and brittle. More recently, some elastomeric materials are becoming available. In the medical world, 3D printer biomaterial selections are even more limited. Although there are a few “FDA friendly,” i.e. biocompatible materials that are starting to be used, we would be hard-pressed to find an actual “bio-friendly” material. Until these material selection limitations are better addressed, it will be difficult for both medical device designers as well as packaging professionals to best utilize the true potential of this technology.
In a now famous example of one of the technologies I have been involved with is the 3D printing of a permanent implant—in this case, a bioabsorbable tracheal splint.ii But the same idea can be applied to all areas of the body. What’s the process? Here’s one possibility: 1) scan the patient via CT or MR, 2) design a “personalized” implant based on the scanned data 3) send the design to the 3D printer in the hospital and 4) implant into the patient. And it’s not a new idea—in some cases, we are actually doing it now!
But like all new technologies, there are many questions to consider including 1) How do we test such devices… if at all? 2) What’s the regulatory pathway to market? In the example cited, an orphan indication (i.e., tracheobronchomalacia in newborns) was chosen. But what about making “custom” devices for the masses? In the spirit of looking for similarities where no similarities seem to exist, this is exactly what happened in the New England Compounding Center case. Is this a combination product? And bioabsorbable materials (i.e., polycaprolactone) present many regulatory challenges! Maybe we will need to create a new regulatory pathway to market such technologies?
For that matter, should FDA regulate this at all? This can be viewed as personalized medicine for medical devices, and like pharmaceutical compounding, it can be argued it is the practice of medicine and therefore outside the jurisdiction of the FDA… at least for now!
In the past, another limitation of 3D printing technology has been physical size, i.e., the larger the part the more expensive to produce, whereas the smaller the part the more difficult to produce. Today, small-scale 3D printing is possible. One example is the 3-D printing of tiny cages to contain bacteria for the control of infection.iii So how long until we can 3D print at the nanoscale? We can even do this now but that’s a topic for another column.
Can we 3D-print new drugs and living tissues? Why not?
I cannot end this column without sharing what I think are the most “wicked cool” application of 3D printing in medical technology—3D printing of drugs and living tissue! You might think this is pure science fiction, but we are already doing it. See “3D Printing Drugs (Tech Feed, June 2013)" ; 3D Printing Could Create Better Pills (in-Pharma Technologist, June, 2013).
Another example is the 3D printing of tiny human livers.iv What is the potential impact of this technology?
Beyond the obvious applications like organ transplants, consider the traditional drug-development model: we do in vitro (benchtop and/or computational) testing) followed by animal testing and ultimately human trials. Although we have been developing drugs like this for decades, does it make sense? Not really. It is an extraordinarily inefficient process resulting in 20% to 50% of drug candidates failing when moving from animal to human testing at a cost of up to $500M!v
More importantly, we assume if a drug is not safe and/or effective in animals, it will not be safe and/or effective in humans. But this is not necessarily so. As a result, how many good products have been scrapped because they didn’t work in animals? So for many reasons, this is a very inefficient process and there has got to be a better way!
What if we modify the model? What if we eliminate animal testing and use the following model: in vitro (benchtop and/or computational testing followed by “engineered human tissue testing” and finally human trials?
Now that we are beginning to 3D print actual living tissues like tiny human livers, this is not nearly as far off as some might think! And it’s an interesting example of the convergence between the medical device and pharmaceutical industries, both benefiting synergistically by improving the drug development process. Who else would be happy with this new drug development paradigm? Those who are not keen on animal testing to begin with, i.e., PETA—the People for the Ethical Treatment of Animals, also known as the People for the Eating of Tasty Animals!
To wrap up, I make many references to Star Trek in my presentations and my columns, including this one. In the late 1960s, many of the devices used in the original Star Trek series were pure science fiction at the time, i.e. floppy disks, cell phones, etc. Today, not only have they become a reality but some have become ubiquitous as well. But some of Gene Roddenberry’s ideas still remain science fiction—or do they?
To most, the idea of the transporter, which can “dematerialize” a person or object and magically “transport” them or it to some far-off place is pure science fiction—or is it?
Consider this: an engineer in the U.S. can design a medical device on a computer and electronically send (or transport) that design to a far-off land like Haiti where a 3D printer can physically recreate the medical device designed by the engineer in the US. Not quite as impressive as the Star Trek transporter but we’re getting there!vi
The goals of personalized medicine for drugs can be summarized as follows: Deliver the correct drug, at the correct dose, at the correct rate, to the correct patient, at the correct time. All of these goals are becoming achievable. The goals for personalized medicine for medical devices are very similar: Deliver the correct device, in the correct size, at the correct shape, to the correct patient, at the correct time. With 3D printing and the other ideas presented here, all of these goals are becoming achievable as well!
Arthur C. Clarke said, “Any sufficiently advanced technology is indistinguishable from magic.”vii To some in this audience, the ideas described in my presentations and columns may seem magical. I would certainly hope so. My philosophy is simple: to set the bar high so that we achieve more. If we continue, as many do, to set the bar so low that we just about trip going over it, then what have we accomplished? In his famous, “We choose to go to the moon” speech Sept. 12 1962, President John F. Kennedy said, “We choose to go to the moon in this decade and do the other things, not because they are easy, but because they are hard, because that goal will serve to organize and measure the best of our energies and skills, because that challenge is one that we are willing to accept, one we are unwilling to postpone, and one which we intend to win.”
The first is to set the bar higher—the next step is to figure out how to get over it —the final step is to go back and repeat step one!
Dr. Michael Drues is president of Grafton, MA-based Vascular Sciences, an education, training, and consulting company. He can be reached on Linkedin®, by phone at 508.887.9486, and by e-mail at [email protected]
References:
i Healthcare Packaging Magazine, Aug. 2013
ii Bioresorbable Airway Splint Created with a Three-Dimensional Printer (NEJM, May, 2013) http://www.nejm.org/doi/full/10.1056/NEJMc1206319.
iii 3-D Printed Microscopic Cages Confine Bacteria In Tiny Zoos For The Study Of Infections (Medical Design On-line, Oct. 7, 2013) http://www.medicaldesignonline.com/doc/d-printed-microscopic-cages-bacteria-tiny-zoos-infections-0001?sectionCode=News&templateCode=Single&user=2128969&source=nl:38432.
iv 3D printer makes tiniest human liver ever (New Scientist, April, 2013) http://www.newscientist.com/article/dn23419-3d-printer-makes-tiniest-human-liver-ever.html.
v Biomaterials for the 21st Century (Robert Langer, Ted Talk, March, 2012)
vi http://www.youtube.com/watch?v=cMzfyGBfAck / Full episode: http://www.youtube.com/watch?v=GDLU7T0U1NY
vii Sir Arthur Charles Clarke, (1917 – 2008) was a British science fiction author, inventor and futurist, famous for his short stories and novels, including 2001: A Space Odyssey.